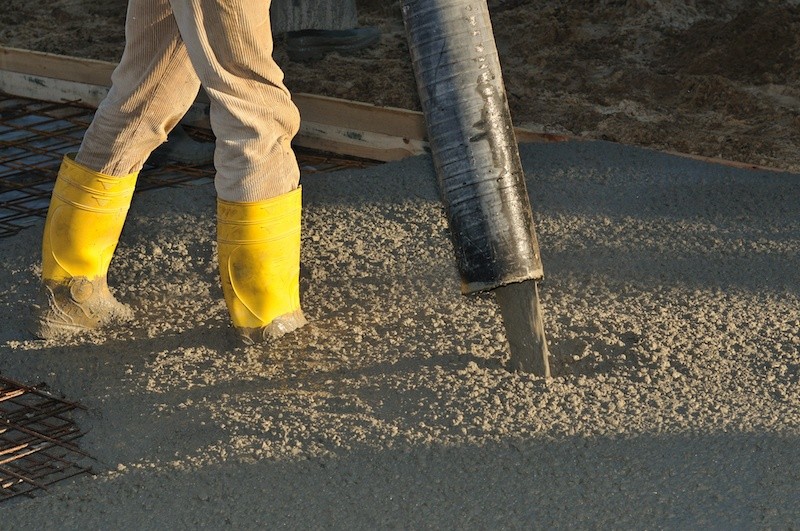
Pressure measurement in abrasive media using Vulkollan® membranes
Typically, pressure sensors are available in either stainless steel or titanium finishes. In this way, all of the common test bench applications or monitoring tasks are covered. But when it comes to contact with particularly abrasive media, then additional protection is required. An added Vulkollan® membrane can often meet the requirements here.
Before turning to two specific application examples, first a few introductory words on the material itself: Vulkollan® is the trade name for a polyester-urethane rubber, a polyurethane plastic with elastic properties, as well as good chemical and mechanical resistance. This rubber-elastic material is used in different variants including foam, cellular soft plastic and also as a solid plastic. While the first two variants are predominantly used in pigging technology, the solid plastic one is processed into wheels, rollers and coatings. The operating temperature range here lies between -20 to +80 degrees Celsius.
Concrete as contact media
A market leader in the field of specialist civil engineering contacted STS in the search for a pressure sensor, which can be used without hesitation in a flowing, abrasive medium. In this particular instance, concrete was the case. The specialist engineer makes hydraulic equipment that drills holes in the ground and then fills them with concrete to form piles.
To ensure that these concrete piles have a stable structure, a continuous flow of concrete must be ensured. The concrete is filled into the hole via a pipe. After the pipe has been inserted into the hole, it can happen that concrete blocks the pipe inner, leading to an interruption of the process.
To prevent this, a pressure sensor was to be inserted into the interior of the pipe. Since the concrete is delivered through the pipe into the drilled hole by means of a pump, a blockage can be easily recognized by high pressure inside the pipe. For this task, a stainless steel pressure sensor was out of the question, since it would survive in concrete only for a short time.
To meet this challenge, STS proposed to furnish a flange sensor fitted with an additional Vulkollan® membrane. With this added protection, the sensor employed achieves a lifespan of one year at a five percent accuracy. The mechanical construction, as well as the electrical connections, are custom designs, which could be supplied within a short time.
Fill-level measurement in trimming tanks
A manufacturer of ship control systems looking for a reliable solution to water-level measurement inside trimming tanks approached STS.
Trimming tanks are used to bias the position of the center of gravity within a vessel. Cargo ships, for example, are constructed in such a way that the design waterline coincides with the actual waterline when fully loaded. But if these are put to sea without cargo, the hull lifts out of the water so much that the bow usually towers way above the water. Because of the weight of the engine, the hull sits deeper, but potentially not deep enough that the propellers are still sufficiently immersed in the water – the ship becomes unable to maneuver in this scenario. To counteract this, the trimming tanks are filled with water.
The pressure transmitters for monitoring the filling level, however, come into contact not only with salt water (where titanium housings are sufficient), but also with sand, small stones or even shells. To optimize the lifetime of the sensor, its membrane was coated with a Vulkollan® film.

Image 1: Example of a pressure transmitter with Vulkollan® foil
It is because of Vulkollan® that pressure sensors can be optimized for use within abrasive media. This does not apply, however, to explosive substances or acids. Read more about the media compatibility of peizoresistive transducers here. In addition, users must remember that this additional Vulkollan® protection adversely affects the precision of the sensor. The thermal characteristics also become more unstable.
As a result, nothing beats the comprehensive and qualified advice of experts in the search for a suitable pressure-measurement solution in abrasive media.